Substitution reactions occur when one functional group replaces another in a molecule. In nucleophilic substitution reactions a nucleophile (Nu), a functional group that is attracted to positive charge, replaces a functional group called a leaving group (LG):
Nu + R-LG → R-Nu + LG
Table of contents:
SN2 reactions
In the SN2 reaction, the addition of the nucleophile and the departure of the leaving group occur in a concerted (taking place in a single step) manner, hence the name SN2: substitution, nucleophilic, bimolecular. In the SN2 reaction, the nucleophile approaches the carbon atom to which the leaving group is attached. As the nucleophile forms a bond with this carbon atom, the bond between the carbon atom and the leaving group breaks. The bond making and bond breaking actions occur simultaneously. Eventually, the nucleophile has formed a complete bond to the carbon atom and the bond between the carbon atom and the leaving group is completely broken.
In the image below, we introduce the concepts of arrow pushing and of a reaction mechanism. Recall that electrons compose the bonds in molecules. Hence for any reaction to occur, electrons must move. In arrow pushing, the movement of electrons is indicated by arrows. Arrows may show electrons forming or breaking bonds or traveling as lone pairs or negative charges on atoms. A complete schematic showing all steps in a reaction, including arrow pushing to indicate the movement of electrons, constitutes a reaction mechanism. In the reaction mechanism below, observe that the electrons from a negative charge on the nucleophile "attack" or form a bond with the carbon atom to which the leaving group is attached. In the center image, the partially formed bond is visiable, as well as the partially broken bond to the leaving group. In the final image, the bond to the leaving group is broken when its electrons become a negative charge on the leaving group.

Figure 1: SN2 reaction showing concerted, bimolecular participation of nucleophile and leaving group
A consequence of the concerted, bimolecular nature of the SN2 reaction is that the nucleophile must attack from the side of the molecule opposite to the leaving group. This geometry of reaction is called back side attack. In a back side attack, as the nucleophile approaches the molecule from the side opposite to the leaving group, the other three bonds move away from the nucleophile and its attacking electrons. Eventually, these three bonds are all in the same plane as the carbon atom (center image). As the bond to the leaving group breaks, these bonds retreat farther away from the nucleophile and its newly formed bond to carbon atom. As a result of these geometric changes, the stereochemical confirguration of the molecule is inverted during an SN2 reaction to the opposite enantiomer. This stereochemical change is called inversion of configuration.
The concerted mechanism and nature of the nuecleophilic attack in an SN2 reaction give rise to several important results:
- The rate of the reaction depends on the concentration of both the nucleophile and the molecule undergoing attack. The reaction requires a collision between the nucleophile and the molecule, so increasing the concentration of either will increase the rate of the reaction.
- Since the unique geometry of back side attack is required, the most important factor in determining whether an SN2 reaction will occur is steric effects. Steric effects refer to the unfavorable intreaction created when atoms are brough too close together. In effect, if the nucleophile or the molecule undergoing attack have too many substituents or substitutents which are too bulky, the reaction cannot occur since the nucleophile will be unable to get close enough to the molecule to do a backside attack.
Substrate effects
Based on the factors described above, we can outline several trends that affect the liklihood of an SN2 reaction occurring. As described above, the most important factor in deciding whether an SN2 reaction occurs is steric effects. When we describe the nature of the molecule being attacked, called the substrate, we can look at how many alkyl substituents are present on the molecule. For example, the molecule may contain a carbon atom attached to a leaving group and three hydrogren atoms. This would constitute a methyl carbon atom, in analogy to methane. Given that such a molecule has almost no steric hindrence (substituents blocking access to the carbon atom), an SN2 reaction will be highly favored in a molecule with a methyl carbon.
We call a carbon atom with one alkyl substituent a primary carbon atom. SN2 reactions will proceed easily with a primary carbon atom, though not as fast as with a methyl carbon. If there are two alkyl substituents, we call the carbon atom secondary. It is possible for an SN2 reaction to proceed at a secondary carbon atom, but it is not as favorable as in the methyl or primary cases. Finally, if there are three alkyl substituents attached to the carbon atom, we have the case of a tertiary carbon atom. In this case, there is far too much steric hindrence, and the SN2 reaction cannot proceed. The principle that increasing substitution leads to decreasing reactivity is outlined in the following table:
|
||||||
Methyl | > | Primary | > | Secondary | > | Tertiary |
The degree of substituion of the molecule undergoing attack is not the only factor that influences the rate of an SN2 reaction. Recall that steric effects (the likelihood that attack by the nucleophile will be blocked) are of paramount importance in determing whether an SN2 reaction will take place. Degree of substitution is one factor in determining steric effects, but it is not the only one. We also need to condisder the bulkiness of the substituents. Consider the following molecule:
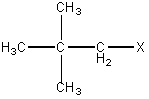
Figure 2: The molecule 1-halo-2,2 dimethylpropane does not undergo substitution
The generic molecule 1-halo-2,2 dimethylpropane is a primary alkyl halide. If we consider only degree of substitution, we would expect this molecule to be capable of undergoing an SN2 reaction. However, the substituent in this case is highly branched and very bulky. Recall that the ability of the nucleophile to attack the carbon atom to which the leaving group is attached is the most important factor in an SN2 reaction. In this case, the bulky, branched substituent means that 1-halo-2,2 dimethylpropane will not undergo an SN2 reaction even though it contains only a primary substituent.
Nucleophile effects
A major factor affecting the SN2 reaction is strength of the nucleophile. Recall that a nucleophile is a molecule which is attracted to positive charge. We can describe several general trends which determine the stregnth of a nucleophile:
- A molecule which has lost a proton and is negatively charged (base) is stronger than the neutral, protonated version of the same molecule (conjugate acid).
- Nucleophilicity of the attacking atom decreases from left to right in the periodic table because atoms because atoms are more electronegative (hold electrons more closely) going from left to right.
- Nucleophilicity of the attacking atom increases down the periodic table as electronegativity decreases and polarizibity increases. Polarizibility refers to the ability of larger atoms lower in the periodic table to share electrons more easily because their electrons are more loosely held.
In addition to the strength of the nucleophile, the structure of the nucleophile also influences the capacity of an SN2 reaction to proceed. As stated many times previously, steric effects are the most important factor in determining whether an SN2 reaction can proceed. We have discussed these effects with regard to the substrate, but they also apply to the nucleophile. A small, unbranched nucleophile will be more effective in an SN2 reaction than a large, branched nucleophile. Consider the following molecules:
![]() |
|
2-methyl-2-propanol | methanol |
The molecules 2-methyl-2-propanol and methanol contain the same attacking atom and the same charge, but 2-methyl-2-propanol is much more sterically hindered than methanol, so it is a much weaker nucleophile.
SN1 reactions
In contrast to an SN1 reaction, in which the bond-making addition of the nucleophile and the bond-breaking departure of the leaving group occur in a single step, the SN1 reaction involves two separate steps: first the departure of the leaving group and then the addition of the nucelophile.
In the SN1 reaction, the bond between the substrate and the leaving group is broken when the leaving group departs with the pair of electrons that formerly composed the bond. As a result, the carbon atom to which the bond was formerly made is left with a positive charge. This positive charge on a carbon atom is called a carbocation, from "carbon" and "cation", the word for a positively charged atom. The formation of a carbocation is not energetically favored, so this step in the reaction is the slowest step and determines the overall rate of the reaction. The step which controls the overall rate of a reaction is called the rate-determing step.
Only after the leaving group has departed and a carbocation has formed, a nucleophile forms a bond to the carbcation, completing the subsitution. This step is more energetically favorable and proceeds more quickly.
There are several important conseuqences to the unimolecular nature of the rate-determing step in the the SN1 reaction. First, since the rate is controled by the loss of the leaving group and does not involve any participation of the nucleophile, the rate of the reaction is dependent only on the concentration of the substrate, not on the concentration of the nucleophile. Second, since the nucleophile attacks the carbocation only after the leaving group has departed, there is no need for back-side attack. The carbocation and its substituents are all in the same plane (Figure 3), meaing that the nucleophile can attack from either side. As a result, both enantiomers are formed in an the SN1 reaction, leading to a racemic mixture of both enantiomers. Finally, since the nucleophile does not participate in the rate-determining step, the strength of the nucleophile does not affect the rate of the SN1 reaction.
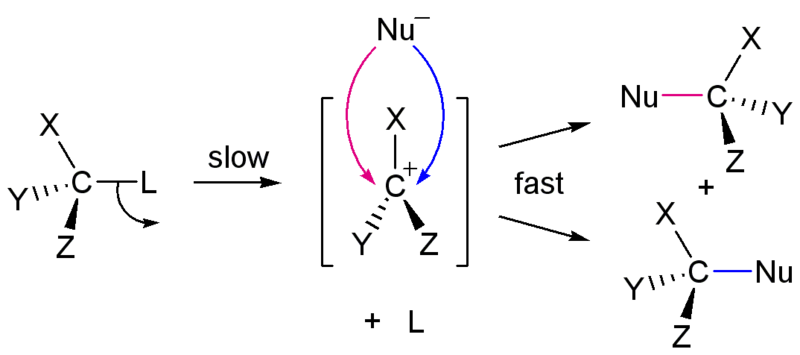
Figure 3: SN1 reaction showing unimolecular step and formation of racemic mixture
What factors govern the rate the probability of an SN1 reaction? The single most important factor is the stability of the carbocation. Alkyl substituents increase the stability of a carbocation, so increasing alkyl substitution of the carbon atom increases the probability of an SN1 reaction occuring.
|
||||||
Tertiary | > | Secondary | > | Primary | > | Methyl |
Recall that, as in the case of an SN1 reaction, the above trend regarding degree of substition is just a trend and the real factor that determines whether an SN1 reaction can occur is the stability of the carbocation. From above, we would expect an SN1 reaction not to occur at the site of a primary carbon atom. Indeed, such reactions do not occur in ordinary alkanes. However, in molecules in which the carbon next to the site of substitution contains a double bond, the SN1 reaction is possible. The reason is that the positive charge on the carbocation can be delocalized among multiple possible resonance structures (see Resonance and delocalization), making the carbocation dramatically more stable. This effect can occur when the carbon atom of interst is next to one double bond (allylic) or a benzene ring (benzylic). Note that in the allylic case, because of the delocalization of the positive charge, the nucleophile can attack at multiple sites (Figure 4); this effect is absent in the benzylic case due to the need to preserve aromaticity. In summary, the key to the SN1 reaction is the stability of the carbocation.
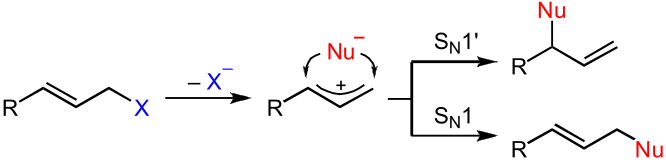
Figure 4: SN1 reaction at a primary carbon atom due to delocalization and resonance
More to come
Previous topic: Aromaticity - benzene |
8. SN2 reaction
Back to index |
Next topic: - |